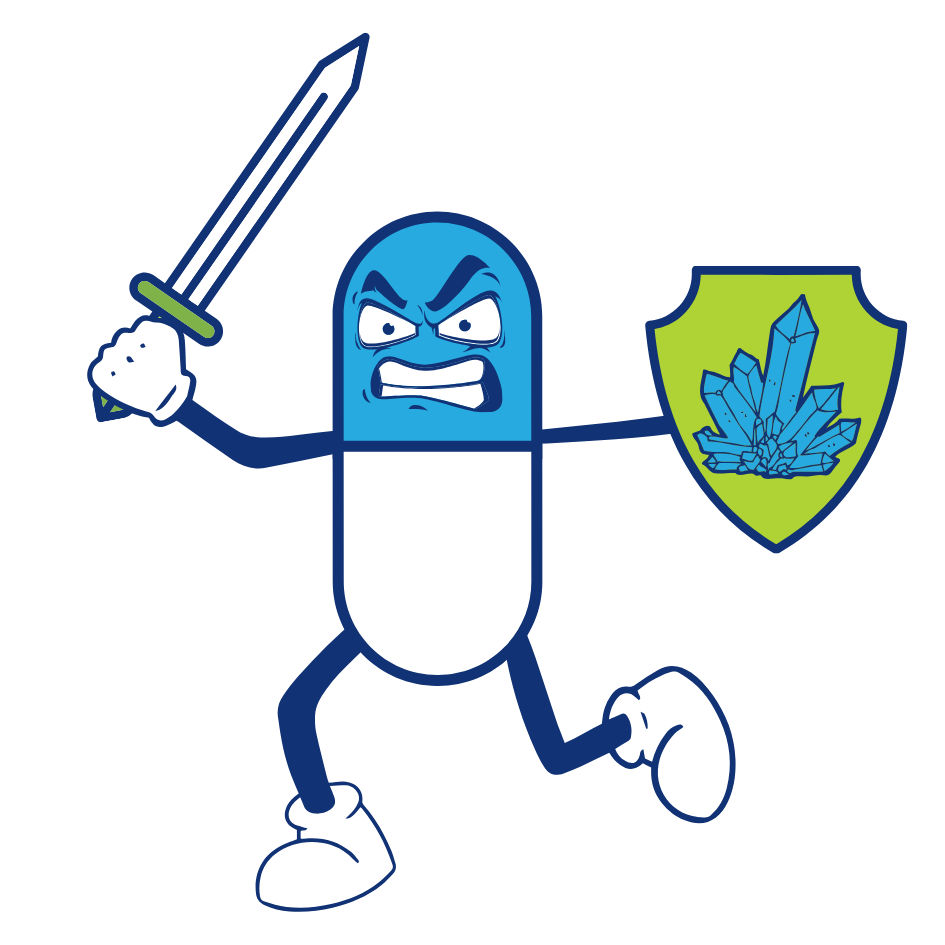
Drug-resistant germs are big killers. The World Health Organization estimates that infections caused by drug-resistant microbes help kill close to five million people a year, a number that’s expected to grow.
The world needs new antibiotics, but bacteria are outwitting scientists and drug developers at every turn.
Microbes can produce complex molecules, such as antibiotics, to protect themselves from other organisms. And they naturally develop survival mechanisms to fight these molecules, including swapping genetic material among themselves. On top of that, continuous exposure from our own use of antibiotics contributes to the inevitable rise of bacteria that can survive even the newest antibiotics.
Among the biggest killers are Gram-negative bacteria, which are also harder to fight because of their extra layers of protection – or armor, as Dr. Skyler Cochrane, a research scholar at Duke University, calls it.
Gram-negative bacteria cause plague, cholera, whooping cough, salmonella, typhoid fever, and urinary tract infections, and are the root of many pneumonia and bloodstream infections.
Cochrane is working in a lab that is looking for chinks in the armor of Gram-negative bacteria.
In this episode of One World, One Health, listen as she talks about a promising new compound that might just offer the first new weapon against these bacteria in decades.
Maggie Fox 00:00
Hello and welcome to One World, One Health with the latest ideas to improve the health of our planet and its people. I’m Maggie Fox. All of us here on planet Earth are facing problems, such as pollution, climate change, and infectious diseases (old and new). Animal health, human health, plant health, and the climate; they’re all linked. This podcast is brought to you by the One Health Trust with bite-sized insights into ways to help.
The world desperately needs new antibiotics, but bacteria are outwitting scientists and drug developers at every turn. Germs live in their own competitive world and they’ve evolved mechanisms to keep rivals and enemies at bay. This makes them naturally resistant to the drugs we, humans, try to develop to fight them. On top of that, our own use of antibiotics leads to the inevitable rise of bacteria that can survive their effects. Labs around the world are working to develop new and better antibiotics, but it’s a hard fight. Most new drugs fail in development. And nowhere is this more true than with antibiotics.
In this episode of One World, One Health, we’re chatting with Dr. Skyler Cochrane, a research scholar at Duke University who is part of a team working on promising new antibiotics, and one that seems to work safely against a range of bacteria. Tests in mice suggest it can kill bugs, such as Staphylococcus aureus, and Klebsiella, which are among the biggest gram-negative killers. We’re hoping to learn a bit more about how difficult it is to develop new antibiotics and what her team has found in the lab. Skylar, thanks so much for joining us.
Skyler Cochrane 01:36
Thanks so much for having me. I’m really excited to be with you guys today.
Maggie Fox 01:39
Can we go back to basics and talk about gram-negative versus gram-positive bacteria? What’s the difference?
Skyler Cochrane 01:48
So, starting with just the name itself, gram-positive versus gram-negative. We’re like, what does this mean? There is a very special dye, it’s a purple color, and it’s called a gram stain. And what happens is when you coat bacteria in this stain, or submerge them in a solution, you’ll either see the bacteria absorb the stain and turn purple, or you’ll see that they don’t have any color change at all. And that’s where gram-positive comes from. It means it absorbs the gram stain. Gram-negative does not absorb it.
And the main reason for this comes down to the actual composition of the cells themselves. So, what makes gram-negative bacteria not absorb the stain and in turn, makes them more difficult for our medicines is the fact that gram-negative bacteria have a double membrane in their cell envelope. But typically, when we think of a cell wall, that’s referred to as peptidoglycan.
And gram-positive bacteria have a thick layer of that along with an inner membrane. But with gram-negative bacteria, that sort of cell wall component is thinner. And then you have another outer membrane sandwiching it, creating this like full cell envelope that’s much more difficult for drugs or foreign substances to penetrate.
Maggie Fox 02:58
And that’s why they’re harder to kill. The drug can’t get in.
Skyler Cochrane 03:02
Gram-negative bacteria are basically wearing an extra suit of armor in comparison to the gram-positive counterparts.
Maggie Fox 03:09
Gram-negative bacteria, as you say, are sometimes naturally resistant to antibiotics just because of their structure and for other reasons as well. But they can also acquire resistance. Can we talk a little bit about the difference between evolved resistance and acquired resistance?
Skyler Cochrane 03:26
Absolutely. So, starting on what you were just saying, we do have like those two basic, there’s intrinsic resistance, which means just the composition of the bacteria itself prevents the drug from working for whatever reason. And then acquired resistance comes in two different forms. And this can either be through a genetic change. So, for instance, the bacteria will evolve some small change somewhere in their DNA that allows them to overcome the bacteria.
So, let’s say you have a protein target and your drug binds to it usually. The bacteria can sometimes evolve a slight change in the composition of that protein, where suddenly there’s something occupying that space or blocking the penetration of the drug from only a single amino acid mutation. And that’s evolved resistance.
Now acquired resistance is kind of fascinating. In that, bacteria can actually transfer their own genes to each other. So, let’s say one bacteria develops a resistance mechanism, it evolves that special little change that we made. It can basically send that little packet of DNA over to other bacteria in the area that can then absorb it and now, they can implement that resistance mechanism as well. It becomes a part of their new DNA.
Maggie Fox 04:34
And, to turn to where antibiotics come from, the the first true antibiotic penicillin came from nature. In that case, it was mold and other antibiotics, such as cephalosporins, came from fungus as well. Bacteria pump out their own antibiotics, but you can also design them in the lab and build them from the ground up. Is that what you’re doing?
Skyler Cochrane 04:56
Yes, absolutely. That is one of the main things that we work on right now. While a lot of current drugs on the market do come from those sort of natural products that we are discussing, it’s kind of hard to identify those sort of new products and identify new ones from nature. So, what we do is there’s so many libraries of millions and millions of compounds and drugs that scientists have isolated and have collected over the years.
And whenever we have a new target that we want to look at, we can do what’s called High Throughput Screening, where you can screen libraries of all of those millions of drugs against your target in a very fast method. And from those, you can get a couple of ones that pop up that have at least some of that. And then, we can look at that base structure, see how it actually works in the enzyme, create a model of it, and then start to make changes using a rational thing. So, if we look and we see our drug occupies this one small pocket, but there’s all this other room around it, we can use our chemical knowledge to think of extra groups that we can add ——. We can extend it to create new little interactions.
One of the ways that I like to think about proteins is like a really, really complicated 3D lock. And our drug design is about crafting the perfect key—starting from one little piece at a time. And the way that we do this is this technique of development called Structure-Activity Relationship, or SAR, which is my favorite method for drug development. It’s got a very logical progression to it. So, where it starts is, you know, we have this compound that we think could work. And what we’ll do is we’ll test it against our purified target on its own, then we’ll test it against the bacteria itself.
So first, we see does it work in our target ——. Next, we’ll test and see can it penetrate the bacteria to get to its target. Though, (can it be) we’ll have efficacy there. And then when we see that our drug is effective in both, we’ll do this technique called X-ray crystallography. And basically, what this is, when we think of things like crystals, we think of (like) diamonds and stones and all of those things. But crystals can actually form out of so many different materials, including compounds and proteins (can form crystals).
So, what I do is I will form crystals of my protein with the drug present. And these crystals are microscopic. They’re grown in a two microliter drop. We have to scoop them under a microscope. I have this little tiny ——. So, my friends refer to me as a crystal cowboy. It’s kind of fun. But once we harvest these crystals, we can ship them off to a facility where they will shoot X-ray beams at the crystal. When crystals form, it’s basically a perfect lattice structure of whatever your material is. So, it will form in this perfect reflective state.
So, when an X-ray beam hits it, it’s going to diffract those beams at different angles and unique ways depending on what your core structure is. So, basically, that beam will hit it and you’ll have a whole room of diffracted X-ray beams with sensors that can pick that up and then reverse engineer an atomic level 3D model of a protein. And you can literally see the presence of your drug in it, in this atomic density.
So, from that, we’re able to create these beautiful crystal ribbon diagrams, where we can see where the drug is present, where it’s interacting, and then use that to make new designs. We send that back to our —— with our idea; they’ll help us synthesize the new drug. And we’ll start the process all over again. And then once we feel like we have good enough properties, we will advance that to further trials. In our case, we go to mouse models.
Maggie Fox 08:14
So, you’re using over and over again this word target, and you have one. Can you tell us a little bit about the target that you’re working on?
Skyler Cochrane 08:21
Yes. So, we are targeting a protein specifically called LpxC. This protein is part of a biosynthetic pathway for a very special component in gram-negative bacteria. So, I was telling you before how gram-negative have a special double membrane and an extra layer of shielding. When we look at that outer membrane itself, the very outermost component of it is formed by something called lipid A. So, lipid A binds together on the very, very outer surface.
You can almost think of it like the epidermis of the bacteria (that outer layer), that if you could touch it, (it) would be what you interact with. Basically, lipid A is the first line of defense that gram-negative bacteria have to drugs. So, the synthetic pathway how the bacteria creates lipid A is a seven-step pathway of different. So, (we’ll start with) it’ll start with a base component. And it will go through each of these proteins.
Basically, they’re little machines. When you have something, it goes into the machine, (and) it comes out slightly different or ——. And —— the pathway itself is a conveyor belt of these different machines. And at the end, you get the final lipid A component that goes out, forms this layer. Our target LpxC is the second step in this pathway. It’s been found that if you can inhibit the production of lipid A, you can cause cell death and many gram-negative bacteria because it will essentially destabilize that outer membrane and contribute to the eventual falling apart of the bacteria.
So, with our target, if we can stop it from working, we can clog up that conveyor belt pathway and the bacteria will eventually die.
Maggie Fox 09:54
And it looks like this compound safely and effectively can kill a range of bacteria. Can you tell us a little bit how it does that and the safety factor as well?
Skyler Cochrane 10:04
Not just a few bacteria, we tested our drug against over 285 different strains, including both resistant and non-resistant strains that have already been identified. So, we found that largely, it is not affected by known resistance mechanisms, which is very exciting. And then, we did a bunch of different safety and efficacy testing of this drug. So, to begin with, usually, once we have a drug that we want to take to some sort of live model testing, we’ll start with different toxicity studies.
So, for instance, we will put mammalian human cells and put them in the presence of the drug, (and) make sure it’s not toxic to human cells to begin with. Next, we’ll start doing studies in mice and rats. So, we did a seven-day toxicology study in rats, where we gave them high doses of the drug just to see would it affect them, would they show any negative side effects. And we found that it was completely safe there. We next moved on to mouse model. So, we tested two different strains of E. coli that have certain drug resistances already.
And what we found was when we dose them for five days, twice a day, we were able to 100% clear the infection from both bacteria in these mice. We repeated the studies using orally administrated or orally given drugs instead of our usual injections. And we found that the drug was just as effective when orally dosed, instead of injected. And why this is important is it’s fine when you’re in a hospital to have to get an injection of a medicine, right. But no one wants to take needles home and have to give themselves shots at home when they’re already feeling sick and having an infection.
So, when it comes to something that can be broadly given to the general population—a drug—you always want it to ideally be orally available, which means you can take it as a pill, and it can be absorbed through your stomach. So, we were really excited to see that our drug worked through both different ways—that it could be absorbed by your body when given that way.
Maggie Fox 11:52
So, let’s just be clear. You have not tested this in people yet.
Skyler Cochrane 11:56
We would ideally like to eventually move to human studies. That is the goal of this work. It still might be a little while before we reach that step, because there’s a lot of other processes involved before that, including formulation, testing, and other things. Because you don’t want to give it to humans until you feel really, really good about it.
So, you got to keep in mind, these are human lives that we’re doing. And medicine is designed to help heal. And we want to make sure that before it goes into a person that we feel the most confident about it that we can.
But, so far from all of our studies, this is the most promising LpxC inhibitor to be produced to reach this sort of stage. There only have been two other inhibitors of our enzyme in history that have made it to clinical trials. But both of them got disqualified early on because they were found to induce cardiovascular toxicity. So, they were causing issues with heart and causing heart damage.
But one thing that we showed in our study that was exciting is we actually did cardiovascular studies because of this known effect. And we found that we had no cardiovascular toxicity and no changes in the heart health with administration of our drugs. So, that means we are the first inhibitor to not induce this toxicity, and to have this high level of efficacy. So, we do hope to eventually progress this to clinical trials. And we hope that we have overcome this obstacle that has been in the way of this target in the past.
Maggie Fox 13:18
But a lot more can go wrong. We know drug testing is hard and most antibiotics fail in testing. What do you see that might go wrong as you go forward?
Skyler Cochrane 13:27
There’s always so much that can go wrong in science. So, there’s only so much that we can test ahead of time that we think of. So, for instance, like I was mentioning with those two previous inhibitors, they didn’t know there would be cardiovascular toxicity before they went to clinical trials. It was something that they didn’t know until they got there. And in the same way, we can’t predict what side effects or off target effects we might have further down the way.
That’s only something that time can tell. But we do have the promising features from our preliminary studies that we are very hopeful (for). And one of the good things for us is since we’re targeting something that is exclusive to gram-negative bacteria, LpxC doesn’t exist in humans. It doesn’t exist in a lot of the places we would be concerned (about). So, as long as our drug, if we can make it (that perfect key that only fit that lock), we’re very hopeful that it won’t have any off-target effects.
Antibiotics are grouped into things called classes and a class of antibiotics are defined by core structure. They’ll all share this very similar center point with just different variations off of them. So, if you think penicillin, amoxicillin, ampicillin, that “cillin” is the reference to the class itself. And they all contain this very specific —— with variations. So, we haven’t had a new class of antibiotics discovered since the 80s (so, in over 40 years). And where that becomes a problem is usually when bacteria develop resistance, they develop class-based resistance.
So, mechanisms that are designed to be resistant to that base feature. So, they’ll work against multiple in that area. So, as we, (are) in hospitals, and we have infections and resistant ones, we keep treating them, but the longer we treat them, the more class resistances these bacteria can build until sometimes (when) we don’t have anything that can work anymore.
Working on these sorts of things, developing this LpxC inhibitor would be a new class of antibiotics should they make it to market or get past clinical trials. So, it would be the first new class of antibiotics to be approved in over 40 years if it makes it that far down the line.
Maggie Fox 15:28
Skyler, thank you.
Skyler Cochrane 15:30
Thank you so much for having me. This has been a joy and a pleasure. I love getting to talk about my research and share more information about science and medicine.
Maggie Fox 15:40
If you liked this podcast, which is brought to you by the One Health Trust, please share it by email or on social media. And let us know what else you’d like to hear about at [email protected]. Thanks for listening.
Maggie Fox 00:00
Hola y bienvenidos a One World, One Health con las últimas ideas para mejorar la salud de nuestro planeta y su gente. Soy Maggie Fox. Todos nosotros aquí en el planeta Tierra enfrentamos problemas como la contaminación, el cambio climático y las enfermedades infecciosas (viejas y nuevas). Salud animal, salud humana, sanidad vegetal y clima; están todos vinculados. Este podcast es presentado por One Health Trust con información breve sobre formas de ayudar.
El mundo necesita desesperadamente nuevos antibióticos, pero las bacterias están burlando a los científicos y a los desarrolladores de medicamentos a cada paso. Los gérmenes viven en su propio mundo competitivo y han desarrollado mecanismos para mantener a raya a rivales y enemigos. Esto los hace naturalmente resistentes a los medicamentos que nosotros, los humanos, intentamos desarrollar para combatirlos. Además de eso, nuestro propio uso de antibióticos conduce al inevitable aumento de bacterias que pueden sobrevivir a sus efectos. Los laboratorios de todo el mundo están trabajando para desarrollar antibióticos nuevos y mejores, pero es una lucha difícil. La mayoría de los medicamentos nuevos fracasan en su desarrollo. Y en ningún otro lugar esto es más cierto que en el caso de los antibióticos.
En este episodio de Un mundo, una salud, conversamos con la Dra. Skyler Cochrane, investigadora de la Duke University, que forma parte de un equipo que trabaja en nuevos antibióticos prometedores, y uno que parece funcionar de forma segura contra una variedad de bacterias. . Las pruebas en ratones sugieren que puede matar insectos como Staphylococcus aureus y Klebsiella, que se encuentran entre los mayores asesinos de gramnegativos. Esperamos aprender un poco más sobre lo difícil que es desarrollar nuevos antibióticos y lo que su equipo encontró en el laboratorio. Skyler, muchas gracias por acompañarnos.
Skyler Cochrane 01:36
Muchas gracias por invitarme. Estoy muy emocionada de estar con ustedes hoy.
Maggie Fox 01:39
¿Podemos volver a lo básico y hablar de bacterias gramnegativas y grampositivas? ¿Cual es la diferencia?
Skyler Cochrane 01:48
Bien, comenzando solo con el nombre en sí, grampositivos versus gramnegativos. Pensamos, ¿qué significa esto? Hay un tinte muy especial, es de color morado y se llama tinción de Gram. Y lo que sucede es que cuando cubres bacterias con este tinte, o las sumerges en una solución, verás que las bacterias absorben el tinte y se vuelven moradas, o verás que no tienen ningún cambio de color. Y de ahí provienen los grampositivos. Significa que absorbe la tinción de Gram. Los gramnegativos no lo absorben.
Y la razón principal de esto se reduce a la composición real de las propias células, lo que hace que las bacterias gramnegativas no absorban el tinte y, a su vez, lo haga más difícil para nuestros medicamentos es el hecho de que las bacterias gramnegativas tienen una doble membrana en su envoltura celular. Pero normalmente, cuando pensamos en una pared celular, se la denomina peptidoglicano.
Y las bacterias grampositivas tienen una capa gruesa junto con una membrana interna. Pero en el caso de las bacterias gramnegativas, ese tipo de componente de la pared celular es más delgado. Y luego hay otra membrana externa que la intercala, creando una especie de envoltura celular completa que es mucho más difícil de penetrar para drogas o sustancias extrañas.
Maggie Fox 02:58
Y por eso son más difíciles de matar. La droga no puede entrar.
Skyler Cochrane 03:02
Las bacterias gramnegativas básicamente llevan una armadura adicional en comparación con las grampositivas.
Maggie Fox 03:09
Las bacterias gramnegativas, como dices, a veces son naturalmente resistentes a los antibióticos simplemente por su estructura y también por otras razones. Pero también pueden adquirir resistencia. ¿Podemos hablar un poco sobre la diferencia entre resistencia evolucionada y resistencia adquirida?
Skyler Cochrane 03:26
Absolutamente. Comenzando con lo que acabas de decir, tenemos esos dos básicos: hay resistencia intrínseca, lo que significa que solo la composición de la bacteria misma impide que el medicamento funcione por cualquier motivo. Y luego la resistencia adquirida se presenta en dos formas diferentes. Y esto puede ser a través de un cambio genético. Entonces, por ejemplo, las bacterias desarrollarán algún pequeño cambio en algún lugar de su ADN que les permita vencer a las bacterias.
Bien, digamos que usted tiene un objetivo proteico y su medicamento generalmente se une a él. A veces, las bacterias pueden desarrollar un ligero cambio en la composición de esa proteína, donde de repente hay algo que ocupa ese espacio o bloquea la penetración del fármaco a partir de una única mutación de aminoácido. Y eso es resistencia evolucionada.
Ahora bien, la resistencia adquirida es algo fascinante. En este sentido, las bacterias pueden transferir sus propios genes entre sí. Entonces, digamos que una bacteria desarrolla un mecanismo de resistencia, desarrolla ese pequeño cambio especial que hicimos. Básicamente, puede enviar ese pequeño paquete de ADN a otras bacterias en el área que luego pueden absorberlo y ahora también pueden implementar ese mecanismo de resistencia. Se convierte en parte de su nuevo ADN.
Maggie Fox 04:34
Y, para abordar el origen de los antibióticos, la penicilina, el primer antibiótico verdadero, surgió de la naturaleza. En ese caso, se trataba de moho y otros antibióticos, como las cefalosporinas, también procedían de hongos. Las bacterias producen sus propios antibióticos, pero también puedes diseñarlas en el laboratorio y construirlas desde cero. ¿Es eso lo que estás haciendo?
Skyler Cochrane 04:56
Si, absolutamente. Esa es una de las principales cosas en las que trabajamos en este momento. Si bien muchos de los medicamentos actuales en el mercado provienen de ese tipo de productos naturales que estamos discutiendo, es un poco difícil reconocer ese tipo de productos nuevos e identificar otros nuevos de la naturaleza. Hay muchas bibliotecas de millones y millones de compuestos y farmacos que los científicos han aislado y recopilado a lo largo de los años.
Y cada vez que tenemos un nuevo objetivo que queremos analizar, podemos hacer lo que se llama Detección de Alto Rendimiento, donde puedes examinar bibliotecas de todos esos millones de farmacos contra tu objetivo con un método muy rápido. Y de ellos, puedes obtener un par de los que aparecen y que tienen al menos algo de eso. Y luego, podemos observar esa estructura básica, ver cómo funciona realmente en la enzima, crear un modelo de la misma y luego comenzar a realizar cambios usando algo racional. Entonces, si miramos y vemos que nuestro medicamento ocupa este pequeño bolsillo, pero hay todo este otro espacio a su alrededor, podemos usar nuestro conocimiento químico para pensar en grupos adicionales que podemos agregar. Podemos ampliarlo para crear nuevas pequeñas interacciones.
Una de las formas en que me gusta pensar en las proteínas es como una cerradura 3D realmente complicada. Y el diseño de nuestro fármaco consiste en crear la llave perfecta, empezando por una pequeña pieza a la vez. Y la forma en que hacemos esto es esta técnica de desarrollo llamada Relación Estructura-Actividad, o SAR, que es mi método favorito para el desarrollo de fármacos. Tiene una progresión muy lógica. Entonces, donde comienza, tenemos este compuesto que creemos que podría funcionar. Y lo que haremos es probarlo contra nuestro objetivo purificado por sí solo y luego lo probaremos contra la bacteria misma.
Primero, vemos si funciona en nuestro objetivo ——. A continuación, probaremos y veremos si puede penetrar las bacterias para llegar a su objetivo. Aunque (puede ser) tendremos exito allí. Y luego, cuando veamos que nuestro fármaco es eficaz en ambos, haremos esta técnica llamada cristalografía de rayos X. Y básicamente, se trata de que cuando pensamos en cosas como cristales, pensamos en diamantes y piedras y todas esas cosas. Pero los cristales en realidad pueden formarse a partir de muchos materiales diferentes, incluidos compuestos y proteínas (pueden formar cristales).
Entonces, lo que hago es formar cristales de mi proteína con el fármaco presente. Y estos cristales son microscópicos. Se cultivan en gotas de dos microlitros. Tenemos que sacarlos bajo un microscopio. Tengo este pequeño —— Por eso mis amigos se refieren a mí como la vaquero de cristal. Es algo divertido. Pero una vez que recolectemos estos cristales, podremos enviarlos a una instalación donde dispararán rayos X al cristal. Cuando se forman cristales, es básicamente una estructura reticular perfecta de cualquier material. Entonces, se formará en este perfecto estado reflexivo.
Cuando un haz de rayos X lo golpea, difractará esos haces en diferentes ángulos y de maneras únicas dependiendo de cuál sea su estructura central. Básicamente, ese rayo lo impactará y tendrás una sala completa de haces de rayos X difractados con sensores que pueden captarlos y luego realizar ingeniería inversa en un modelo 3D a nivel atómico de una proteína. Y puedes ver literalmente la presencia de tu droga en él, en esta densidad atómica.
Entonces, a partir de eso, podemos crear estos hermosos diagramas de cintas de cristal, donde podemos ver dónde está presente el fármaco, dónde interactúa y luego usarlos para crear nuevos diseños. Se lo enviamos de vuelta a nuestro —— con nuestra idea; Nos ayudarán a sintetizar el nuevo fármaco. Y comenzaremos el proceso de nuevo. Y luego, una vez que sintamos que tenemos propiedades lo suficientemente buenas, pasaremos a realizar más pruebas. En nuestro caso nos vamos a los modelos de ratón.
Maggie Fox 08:14
Entonces, estás usando una y otra vez esta palabra objetivo, y tienes uno. ¿Puedes contarnos un poco sobre el objetivo en el que estás trabajando?
Skyler Cochrane 08:21
Sí, nos dirigimos a una proteína llamada específicamente LpxC. Esta proteína forma parte de una vía biosintética de un componente muy especial de las bacterias gramnegativas. Les decía antes que los gramnegativos tienen una doble membrana especial y una capa adicional de protección. Cuando miramos esa membrana externa, su componente más externo está formado por algo llamado lípido A. Entonces, el lípido A se une en la superficie muy, muy externa.
Casi puedes pensar en ello como la epidermis de las bacterias (esa capa externa), que si pudieras tocarla, sería con lo que interactuarías. Básicamente, el lípido A es la primera línea de defensa que tienen las bacterias gramnegativas ante los fármacos. Entonces, la vía sintética mediante la cual las bacterias crean el lípido A es una vía diferente de siete pasos. Entonces (comenzaremos con) comenzará con un componente base. Y pasará por cada una de estas proteínas.
Básicamente, son pequeñas máquinas. Cuando tienes algo, entra en la máquina y sale ligeramente diferente o ——. Y… el camino en sí es una cinta transportadora de estas diferentes máquinas. Por ultimo, se obtiene el componente lípido A final que sale y forma esta capa. Nuestro objetivo LpxC es el segundo paso en este camino. Se ha descubierto que si se puede inhibir la producción de lípido A, se puede causar la muerte celular y de muchas bacterias gramnegativas porque esencialmente desestabilizará esa membrana externa y contribuirá al eventual desmoronamiento de las bacterias.
Entonces, con nuestro objetivo, si podemos evitar que funcione, podemos obstruir el camino de la cinta transportadora y las bacterias eventualmente morirán.
Maggie Fox 09:54
Y parece que este compuesto puede matar de forma segura y eficaz una variedad de bacterias. ¿Puedes contarnos un poco cómo lo hace y también el factor de seguridad?
Skyler Cochrane 10:04
No solo unas pocas bacterias, probamos nuestro medicamento contra más de 285 cepas diferentes, incluidas cepas resistentes y no resistentes que ya han sido identificadas. Entonces, descubrimos que, en gran medida, no se ve afectado por mecanismos de resistencia conocidos, lo cual es muy interesante. Y luego, hicimos varias pruebas diferentes de seguridad y eficacia de este medicamento. Bien, para empezar, por lo general, una vez que tenemos un medicamento que queremos llevar a algún tipo de prueba en modelo vivo, comenzamos con diferentes estudios de toxicidad.
Por ejemplo, colocaremos células humanas de mamíferos y las pondremos en presencia del medicamento, (y) para empezar, nos aseguraremos de que no sea tóxico para las células humanas. A continuación, comenzaremos a realizar estudios en ratones y ratas. Hicimos un estudio toxicológico de siete días en ratas, donde les dimos altas dosis del medicamento solo para ver si les afectaba y si mostraban algún efecto secundario negativo. Y descubrimos que allí era completamente seguro. Luego pasamos al modelo de raton, asi es que, probamos dos cepas diferentes de E. coli que ya tienen ciertas resistencias a los medicamentos.
Y lo que encontramos fue que cuando les administramos dosis durante cinco días, dos veces al día, pudimos eliminar al 100% la infección de ambas bacterias en estos ratones. Repetimos los estudios utilizando fármacos administrados por vía oral o farmacos por vía oral en lugar de nuestras inyecciones habituales. Y descubrimos que el fármaco era igual de eficaz cuando se administraba por vía oral, en lugar de inyectarse. Y la razón por la que esto es importante es que está bien cuando estás en un hospital tener que recibir una inyección de un medicamento, ¿verdad? Pero nadie quiere llevarse agujas a casa y tener que ponerse inyecciones en casa cuando ya se siente enfermo y tiene una infección.
Entonces, cuando se trata de algo que se puede administrar ampliamente a la población general (un medicamento), lo ideal es que siempre esté disponible por vía oral, lo que significa que se puede tomar en forma de pastilla y se puede absorber a través del estómago. Entonces, nos emocionó mucho ver que nuestro medicamento funcionaba de ambas maneras diferentes: que el cuerpo podía absorberlo cuando se administraba de esa manera.
Maggie Fox 11:52
Entonces, seamos claros. Aún no lo has probado en personas.
Skyler Cochrane 11:56
Lo ideal sería pasar algún tiempo a los estudios en humanos. Ese es el objetivo de este trabajo. Puede que todavía pase un poco de tiempo antes de que lleguemos a ese paso, porque hay muchos otros procesos involucrados antes de eso, incluida la formulación, las pruebas y otras cosas. Porque no quieres dárselo a los humanos hasta que te sientas muy, muy bien al respecto.
Debes tener en cuenta que lo que estamos haciendo son vidas humanas. Y la medicina está diseñada para ayudar a sanar. Y queremos asegurarnos de que, antes de que llegue a una persona, nos sintamos lo más seguros posible.
Pero, hasta ahora, según todos nuestros estudios, este es el inhibidor de LpxC más prometedor producido para alcanzar este tipo de etapa. Sólo ha habido otros dos inhibidores de nuestra enzima en la historia que han llegado a ensayos clínicos. Pero ambos fueron descalificados desde el principio porque se descubrió que inducían toxicidad cardiovascular. Entonces, estaban causando problemas cardíacos y daños cardíacos.
Pero una cosa interesante que demostramos en nuestro estudio es que en realidad hicimos estudios cardiovasculares debido a este efecto conocido. Y descubrimos que no tuvimos toxicidad cardiovascular ni cambios en la salud del corazón con la administración de nuestros medicamentos. Entonces, eso significa que somos el primer inhibidor que no induce esta toxicidad y que tiene este alto nivel de eficacia. Por lo tanto, esperamos que eventualmente esto avance hacia los ensayos clínicos. Y esperamos haber superado este obstáculo que ha impedido alcanzar este objetivo en el pasado.
Maggie Fox 13:18
Pero muchas más cosas pueden salir mal. Sabemos que las pruebas de detección de drogas son difíciles y que la mayoría de los antibióticos fallan en las pruebas. ¿Qué ves que podría salir mal a medida que avanzas?
Skyler Cochrane 13:27
Siempre hay muchas cosas que pueden salir mal en la ciencia. Por lo tanto, pensamos que hay muchas cosas que podemos probar de antemano. Entonces, por ejemplo, como mencioné con esos dos inhibidores anteriores, no sabían que habría toxicidad cardiovascular antes de ir a los ensayos clínicos. Era algo que no supieron hasta que llegaron allí. Y de la misma manera, no podemos predecir qué efectos secundarios o efectos fuera del objetivo podríamos tener en el futuro.
Eso es algo que sólo el tiempo puede decir. Pero sí tenemos características prometedoras de nuestros estudios preliminares de las que tenemos muchas esperanzas. Y una de las cosas buenas para nosotros es que, dado que nos dirigimos a algo que es exclusivo de las bacterias gramnegativas, la LpxC no existe en los humanos. No existe en muchos de los lugares que nos preocuparían, mientras nuestro medicamento, si podemos producirlo (esa llave perfecta que solo encaja en esa cerradura), tenemos muchas esperanzas de que no tenga ningún efecto no deseado.
Los antibióticos se agrupan en cosas llamadas clases y una clase de antibióticos se define por su estructura central. Todos compartirán este punto central muy similar con solo diferentes variaciones. Si piensas en penicilina, amoxicilina, ampicilina, esa “cilina” es la referencia a la clase misma. Y todos contienen esto muy específico, con variaciones, no hemos descubierto una nueva clase de antibióticos desde los años 80 (es decir, en más de 40 años). Y donde eso se convierte en un problema suele ser cuando las bacterias desarrollan resistencia, desarrollan resistencia basada en clases.
Mecanismos que están diseñados para ser resistentes a esa característica básica, trabajarán contra múltiples en esa área. Entonces, como estamos en hospitales y tenemos infecciones y infecciones resistentes, seguimos tratándolas, pero cuanto más las tratamos, más resistencias de clase pueden desarrollar estas bacterias hasta que ya no tenemos nada que pueda funcionar.
Trabajando en este tipo de cosas, desarrollar este inhibidor de LpxC sería una nueva clase de antibióticos en caso de que llegue al mercado o supere los ensayos clínicos. Por lo tanto, sería la primera nueva clase de antibióticos aprobados en más de 40 años si llega tan lejos en el proceso.
Maggie Fox 15:28
Skyler, gracias.
Skyler Cochrane 15:30
Muchas gracias por invitarme, ha sido una alegría y un placer, me encanta poder hablar sobre mi investigación y compartir más información sobre la ciencia y la medicina.
Maggie Fox 15:40
Si le gustó este podcast, presentado por One Health Trust, compártalo por correo electrónico o en las redes sociales. Y háganos saber qué más le gustaría saber en [email protected] . Gracias por su atención.
Guest
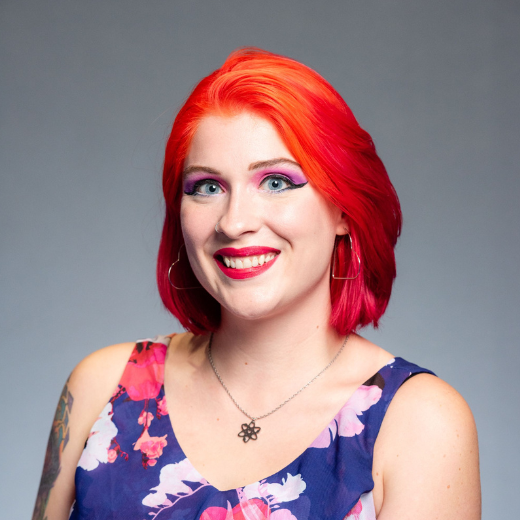
Dr. Skyler Cochrane is a recent doctoral graduate from the Department of Chemistry at Duke University. Hailing from Nashville, Tennessee, Skyler has been working in research labs geared toward medicinal development since she was in high school, and has recently found great success working with Dr. Pei Zhou on developing novel antibiotics geared towards addressing the ever-growing antibiotic resistance crisis. Her passion for and dedication to research have resulted in numerous co-first author publications in respected journals and earned her the Jo Rae Wright Fellowship for Outstanding Women in Science from Duke University in 2021.
Credits
Hosted and written by Maggie Fox
Special guest: Skyler Cochrane
Produced and edited by Samantha Serrano
Music composed and sound edited by Raquel Krügel
Transcript edited by Dipyaman Sengupta